Shade Avoidance and Phase Change in Blackwoods
Wednesday, March 12, 2014, Ian Brown's Blog
When blackwoods grow in natural forests, their form is enhanced by competition for light, but at the cost of slow growth compared to open grown trees. Companion planting is often used in silvicultural systems designed to improve form. This is technically demanding, and has resulted in some impressive failures. In these notes I discuss some of the mechanisms involved in shade avoidance responses, and a possible linkage to phase change in blackwood. Finally, I suggest possible avenues for research, including methods that might induce shade avoidance responses without the need for companion planting.
Shade Avoidance
For a young tree, engaged in a life or death struggle against competing species, survival depends on the capture of light. To achieve this, plants have evolved a mechanism that allows them to detect the presence of neighbouring vegetation. This activates a growth response designed to head off the competitors in the pursuit of light (1).
This process, known as the Shade Avoidance Syndrome, has been the subject of extensive research over several decades. Although supported by an agenda driven by horticultural interests rather than forestry, it is closely linked to plantation and continuous cover forestry. What is emerging from these studies is a complex and fine-tuned process that dates from an early stage of plant evolution, and although differing in detail, has common features across a range of plant species.
Detection
The process starts when light enters a tree canopy, where chlorophyll in the leaves extracts photons in the red and blue bands in the light spectrum in order to provide the energy for photosynthesis. As light passes through the canopy it therefore carries a signature imprinted in the spectrum that is specific for chlorophyll . Neighbouring plants have a mechanism for recognising that signature, and modifying their growth response to optimise light capture. This is the Shade Avoidance Response (SAR ). A key factor in initiating the SAR is a protein pigment, Phytochrome B, which is located in the shoots and leaves of the tree.
Interpreting the signal
Phytochrome B is the most important of several light receptors involved in SAR. In simple terms, this is how it is done.The molecule has three functional components: a protein, a hinge, and a pigment. The protein comprises two chains of amino acids of unequal length. Each is folded into a specific and complex 3 dimensional pattern, the shape of which is determined by its amino acid sequence. The two chains are linked by a chemical hinge, and the hinge is controlled by the pigment.
The role of the pigment is to identify a gap in the red band of the light spectrum. It does so by comparing the ratio of light in the red band with the far red band in the spectrum. A low R/Fr ratio is the signature for chlorophyll, and indicates that another plant in close vicinity is engaged in photosynthesis, and is therefore a potential threat. On the other hand, a high R/Fr signal indicates that the coast is clear, and unmodified light is reaching the plant. Depending on the R/Fr ratio, a signal is transmitted to the hinge, and as this opens or closes, the protein chains move in relation to each other. Phytochrome B therefore adopts either of two forms, Pr and Pfr, each with its own distinctive geometry. Both forms exist in equilibrium, the balance between them determined by the quality of light they receive. Pfr is the active form, and triggers the genetic and chemical pathways that result in the Shade Avoidance Response.
The Shade Avoidance Response
The principal changes that characterise the Shade Avoidance Response are as follows:
- increase in stem height at the expense of diameter
- increase in apical dominance
- reduction in branching
- increase in internode spacing between branches
- reduction in the ratio of roots to shoots
Clearly the diversion of the tree's resources into height growth introduces a conflict of interest between height growth and stability, but this is a contest in which the pursuit of light takes precedence.
Shade avoidance responses have been embedded in the genome of trees over a long evolutionary history. Natural selection has ensured that the intensity of the response varies between species in a manner that matches their ecological niche in relation to shade.
SAR and Shade Tolerance
SARs are most strongly developed among light-demanding pioneer species. This includes most of the fast growing species favoured in plantation forestry. SARs are weaker among the slower growing shade -tolerant species that appear later in forest succession. This effect has been studied in New Zealand in a trial of three species with which we are very familiar: rimu, Kauri, and radiata pine (2). As expected, rimu, which can linger for many years under shade, showed the weakest response. Kauri, which performs well under 50% shade in its juvenile growth, showed a moderate response, and light-demanding radiata pine showed the strongest response.
For rimu and kauri, the SAR matches their ecological niche when competing among canopy species, and receiving modified light from above. However radiata pine requires another explanation. Its natural ecology is closely linked to fire, and in their natural environment radiata seedlings germinate in a barren and ash-strewn landscape, where competition is provided by a swarm of radiata seedlings. After fire, there is no canopy to transmit modified light.
Early studies on SAR were focussed on the effect of canopy shading. Subsequent studies have shown that a phytochrome response does not require a live canopy, but can be triggered by light reflected horizontally from adjacent vegetation (3). It appears that relatively small changes in R/Fr can cause a vigorous phytochrome response, and that this effect can occur at a surprising distance. In a study on poplar, the area most receptive to horizontally reflected light appeared to be located in the stem rather than in the leaves (4). The detection of reflected light is therefore a pre-emptive response to anticipated rather than actual shading.
For radiata pine, a vigorous SAR is triggered by reflected light from other pine seedlings in the intense competition that follows germination after fire. The same is likely to be true for blackwood, where regeneration is linked to fire, and competition is provided by shrubs that are also fire-adapted, but short-lived.
SAR and Provenance
In a study on Acacia implexa, which is closely related to A. melanoxylon, the SAR was found to be strongest in trees from high rainfall populations than from low rainfall populations (5). This might be expected, as in high rainfall locations regeneration is vigorous and competition for light more intense. This is likely to be also true for blackwood, and strengthens the case for selecting blackwood for plantation from high rainfall provenances.
SAR and the Circadian Clock
The strength of shade avoidance responses varies at different times of the day, and is linked to the circadian clock (6). The response is strongest when trees are exposed to modified light at dusk. This might have practical value when trees are interplanted with rows of a faster growing nurse species. In theory a stronger response might be expected from a North/South row orientation, which would capture modified light at dusk.
Friend and foe
When responding to a competitive threat, the SAR does not allow a tree to distinguish between its own branches and those of its neighbours. In other words it cannot distinguish between friend and foe. This is apparent in two situations:
Coppice. When blackwoods are coppiced, several shoots appear from the cut stem. These interact and induce a shade avoidance response, as if they were separate plants. The stems are straight, and show apical dominance and controlled branching. We can take advantage of this by delaying thinning, and allowing the shoots to compete. When they reach a height of 5 to 6 metres, they can be thinned to one stem, and the crown can then be allowed to expand.
Crown competition. When thinning is delayed in a blackwood plantation, the leading branches compete, and form multiple stems. These interact, and develop a shade avoidance response. The live crown then retreats, and is supported by long slender branches. The tree is now condemned to slow diameter growth, and a long rotation. Clearly a SAR is useful during the development of a 5 to 6 metre stem, but should be discouraged during crown development. This can be achieved by timely thinning.
Blue Light Detection
Both red and blue bands in the light spectrum are involved in the SAR. Of these the red band is more important, but the blue band also has a role. Reduction of blue light is detected by another pigment, cryptochrome 1. It has been shown in A. implexa that a reduction in blue light results in stem lengthening, and a reduction in root mass (5).
Phase Change
In blackwood, the transition between juvenile and adult phases of growth is normally apparent within the first two years. Its most obvious feature is the contrast between the fern-like juvenile leaves and the adult phyllodes. However there are other changes that occur during phase transition.
Juvenile Features
1. Leaves The juvenile leaves are adapted to take advantage of low light conditions
- they have a high photosynthetic capture area in relation to their weight (6)
- they lie horizontally, which allows them to capture light from above
- in contrast to phyllodes, they are very efficient in photosynthesis at low light intensity. At higher light intensity, the phyllodes develop higher photosynthetic rates, and juvenile foliage becomes less efficient (10).
- they have an extended annual growth period, a feature shared by juvenile foliage across a range of tree species.
2. Periodicity During the juvenile phase, growth of the terminal shoot is continuous, and this can allow significant height extension. When the trees shift into an adult growth pattern, shoot growth becomes intermittent. From the second year open grown blackwoods commonly show two annual growth periods, the first terminating in December, and the second in early autumn. At the completion of these periods, growth is interrupted by abortion of the shoot tip ( this is common among leguminous species ).When growth resumes, the former leader is replaced by sub-terminal shoots, and the trees adopt a branching habit. Increasing periodicity with age is common across a range of tree species.
3. Architecture Juvenile growth is characterised by:
- strong apical dominance
- long thin stems
- small horizontal branches
- long internodes between branches
- a low root to shoot ratio
4. Resistance to psyllid insects Juvenile leaves have greater resistance to attack by acacia psyllids than the foliage on adult shoots.
Timing of Phase Change
A distinctive contrast between juvenile and adult foliage (heterophylly ) is seen in a range of species (8). It occurs in several of the acacias, and is common among New Zealand native species, where the juvenile plants often have a divaricating structure. In New Zealand species the timing of foliar change is commonly fixed, and is in large part genetically determined. In contrast, among Australian species including the acacias, the timing is plastic, and is more strongly influenced by environmental factors.
Genetic. It has been shown that blackwoods from high rainfall coastal provenances retain their juvenile foliage longer than inland provenances (9). This is likely to be an adaptation to the intense competition for light among species in those favourable growing sites. Provenances from dry inland sites show early phase change, providing the trees with phyllodes that are adapted to hot dry conditions. Moreover, early maturation and seed production would be supported by natural selection in provenances from inland locations which have an increased fire frequency.
Environmental. The chief influences appear to be environmental stress, and light.
Stress. Blackwoods show early phase change on sites that are hot, dry, or exposed to wind. They retain their juvenile foliage longer in warm, moist, sheltered gullies. Another factor causing early phase change could be root disturbance. I have an impression that the juvenile growth phase is extended when blackwoods grow naturally from roots or ground-stored seeds, rather than from transplanted seedlings. This suggests that accelerated phase change could be a non-specific response to a range of environmental stresses.
Light. Blackwoods that grow under a canopy show a delay in phase change. This is likely to be a response to some aspect of light - either a reduction in light intensity, or a modified light spectrum.
Phase Change and the SAR
The attributes that characterise the juvenile growth phase have evolved to give the tree a competitive advantage in the pursuit of light. It will be clear that in growth habit and architecture these closely resemble the changes that are present in the Shade Avoidance Response. This suggests that the two processes might be closely linked, perhaps responding to the same signals, and sharing common mechanisms.
An edge experiment
In 1995, while walking on our Pirongia property in a clearing bordered by 3 metre scrub, and containing blackwoods planted 3 years previously. I noticed that the trees adjacent to the margins were taller, more apically dominant, and retained some juvenile foliage. Those away from the margins had converted to adult foliage.
My son Martin, who was studying science at the University of Waikato, and his tutor Dr John Bathgate made a detailed study of the trees on this site, and in two similar clearings. The results were published in an AMIGO newsletter (12), and can be summarised:
- the trees adjacent to the edge of the clearings were taller than the others. The highest growth was at 1 metre from the edge, and the effect was lost at 3 metres.
- the trees adjacent to the scrub had stronger apical dominance, longer internode gaps, and smaller and more horizontal branches.
- in the stems there were fewer of the collar scars that indicate periodic interruption of shoot growth, indicating that growth had been continuous rather than periodic.
- the taller trees retained juvenile foliage. There was a close relationship between tree height and the proportion of juvenile to adult foliage.
- there was no clear linkage in these effects to shelter from wind, soil variation, or light intensity.
- in summary, the edge trees showed features consistent with both a shade avoidance response, and sustained juvenile growth. We considered a possible response to the light spectrum. A spectrophotometer was borrowed from another project, but encountered technical problems. As events transpired, this may have been unfortunate.
Supporting the linkage.
In a recent study on SAR in Acacia implexa, an Australian researcher, J Forster, examined the response to a low R/Fr signal (5). A. implexa is closely related to A.melanoxylon, and the effect could be expected to apply to both species. This showed a "novel finding", in which the transition from juvenile to adult foliage was delayed by exposure to low R/Fr light.
In a preliminary study, I have looked at the effect of shelters on A. melanoxylon in a small number of trees. These were grown from the roots of the same parent tree and were therefore genetically identical. A group in which shelters were made of a low R/Fr filter showed sustained juvenile foliage compared to controls planted in clear shelters, which converted to phyllodes. The numbers were too few to be significant, and I am planning to extend the trial later this year.
Foolish rice
In the early years of the last century, Japanese rice farmers were confronted with a familiar problem. Their rice crops, which should have been growing into sturdy plants with abundant seeds, had bolted, and were growing into tall spindly plants, and were bending over. By general agreement among the farmers this was an unseemly and inconsiderate form of behaviour. The plants could only be considered as foolish. So this became known as the Foolish Rice Syndrome.
In 1926 a Japanese scientist identified the culprit, a fungal pathogen that had infected the plants. He later identified the causative agent in the fungus, a hormone subsequently named giberellin.
The giberellins
The giberellins are a family of hormones that are involved in a range of physiological processes in plants. These include stem lengthening, breaking seed dormancy, and delaying leaf senescence. They have also been known for some time to be implicated in phase change , in which they delay the transition from juvenile to adult leaves (11).
Testing Giberellin
In 1996, my daughter Nicola was studying science at the University of Waikato. As an adjunct to a study on the effect of different pruning intensities on blackwood we thought it might be of interest to examine the impact of giberellin applied as a spray on a small subset of trees. Might this delay phase change, and if so replicate the growth habit of juvenile trees?
The numbers were small but it looked interesting, and in the following year we set up a formal trial of 120 seedlings, including controls, and applied giberellin (GA3) by stem injection in a range of concentrations from a low dose to what was predicted to be a level of toxicity. The trees reverted to juvenile foliage, and showed an increased height growth. However that is where the good news ended. The trees showed no apical dominance, and the branches grew long and slender, and bent over. Like vegetable plants, also responding to giberellin signals, the trees had "bolted". I felt some sympathy with the Japanese rice farmers. What we had produced was a crop of "foolish blackwoods".
If giberellin was involved in regulating juvenile growth there were clearly other factors involved. Another hormone perhaps, linked to the control of apical dominance.
Giberellin and stem lengthening
Within the last two decades, extensive research has clarified the way in which hormones are integrated into the signalling network in the Shade Avoidance Response. One of these pathways involves the role of giberellin in stem lengthening (13). In summary, this is how it is done:
As light is reflected from a neighbouring plant, Phytochrome B responds to a low R/Fr signal by converting into its active form. Phytochrome enters the cell nucleus where it becomes linked to transcription factors (PIF 4 and 5). These activate genes that code for giberellin synthesis.. Following a sequence of messages from the nucleus and chemical responses within the cell, the plant now manufactures giberellin.
At its destination, comprising cells within the growing stem, giberellin acts by targeting and breaking up a specialised group of proteins referred to as DELLA proteins. These are attached to and hold in check another group of transcription factors which are now unleashed, and they activate a group of genes that code for proteins referred to as expansins. The expansins relax the membranes of cells in the stem, the cells absorb water and stretch, and the stem lengthens.
Missing links
This might explain part of the mechanism of the SAR, but clearly additional pathways are involved. In particular, the apical dominance that characterises both SAR and the juvenile growth phase would need to be explained. If we are looking for another hormone, there is an obvious candidate.
Auxin is the first recognised and best known of the plant hormones. Like the giberellins it controls a number of plant processes, and is commonly used as a rooting hormone. Auxin that is synthesised in the shoot tips has a key role in the control of apical dominance, supporting extension growth of the terminal shoot, and reduction in branches.
The first reports on the part played by auxin in SAR began to appear in the literature in about 2000. Extensive research carried out within the last decade has shown that it has a critical role in that process. Its mechanism of action is complex. It acts indirectly through an influence on giberellin synthesis and pathways. It has a direct effect on DELLA proteins. In addition it has a separate pathway that is independent of giberellin, and this is currently under investigation (13).
Other hormones are now known to be involved. Ethylene has been shown to have a part in SAR. It does so by interacting with and reinforcing the effect of auxin. ( Ethylene has another interesting role. When plants are partially submerged in water , they show a growth response that closely resembles SAR, and here the principal agent involved is ethylene. For a plant, getting its head out of water is as critical for survival as getting it out of the shade. The genes involved in this process have been identified, and are referred to as SNORKEL genes ).
The brassinosteroids are agents of signal transfer in responses to both red and blue light.
Another group, the cytokinins, have an antagonistic effect on SAR. They hold SAR in check, but are inactivated in shade.
As researchers in a range of disciplines piece together the elements in the puzzle that is shade avoidance, what is emerging is a process of great complexity, involving a network of signalling pathways in which light is intercepted and interpreted, genes are activated, hormones act as messengers, and complex proteins are manufactured. In retrospect, when we sprayed giberellin on our trees it was as if we were adjusting the mechanism of a Swiss watch by hitting it with a hammer. In mitigation, most of the current knowledge of SAR and its hormone messengers has emerged since then.
Exploiting shade avoidance
Most of the research on SAR has been driven by an agenda related to horticulture. When growing crop plants, where the aim is to produce short stocky plants loaded with seeds, shade avoidance responses are a problem. The practical application of this work is therefore the suppression of SAR. In contrast, in forest silviculture, shade avoidance responses can be useful in improving the growth and form of trees. Blackwood offers a good example.
Traditional blackwood silviculture might be defined as the manipulation of shade avoidance responses. A SAR, induced by companion planting, is helpful when producing a straight stem of 5 to 6 metres (the same effect can be produced by regular form pruning, but that is another story). On the other hand, as the crown develops above it, a shade avoidance response is undesirable, and will produce a high crown, and condemn the tree to slow diameter growth. This can be avoided by timely thinning.
The use of companion species as a strategy for inducing a SAR is not without problems, and requires close attention to detail. The difficulty lies in reconciling a conflict: to allow enough light for an optimal growth rate, yet with sufficient proximity to induce a shade avoidance response, and to maintain this dynamic equilibrium over several years. Added to this is the competition between crop and nurse species for soil resources of nutrients and water.
There might be an alternative and simpler method for dealing with these conflicting interests. This could involve the use of modified tree shelters.
Tree Shelters
Tree shelters give a stimulus to growth by providing warmth, humidity, and protection from wind. In a study using 60 cm. KBC shelters on blackwoods I measured a 25% increment in height in the first summer compared with controls (15). When using 1.2 m. shelters, the height increment was greater.
KBC shelters are designed to encourage strong growth by transmitting a natural light spectrum, with a high R/Fr ratio. However for blackwood, or other species where a shade avoidance response is desirable, there could be a case for designing a shelter which filters out red light, and transmits a low R/Fr spectrum. A UK firm, Lee filters, produce a range of light filters ( these are presented in an excellent website which illustrate the light spectrum in a range of colours). Those that most closely match a low R/FR ratio are in the green and blue range of colours. Of these, Lee 121 would seem one of the most suitable.
I have constructed shelters of Lee 121 filters, and tested them on a small group of blackwoods that are genetically identical. These have shown a strong retention of juvenile foliage compared with trees sheltered in clear plastic, and open grown trees. I think this would be worth testing in a more formal trial. The Lee filters are light, and only suitable for experimental purposes, but if a useful response could be demonstrated, more robust shelters could be produced. My suggestion would be a height between .6 and 1.2 metres.
In theory, some problems might occur with this type of shelter:
- a reduction of photosynthetically active light in the red band might cause a reduction in growth rate. However this was not apparent in a preliminary study. Moreover, Lee 121 shelters transmit 35% red light, which is unlikely to be a limiting factor for growth, and as referred to previously, juvenile foliage is photosynthetically active in low light intensity.
- the trees might be unstable when they emerge from the shelters. This has not been a problem with blackwoods grown in KBC shelters. Instability is a problem if shelters are used with softwood species, such as cypress (16).
- the effect might not be sustained after the shoots emerge from the shelters.
To maintain a response, a continued exposure to low R/Fr light might be supported by group planting.
Group Planting
When using companion planting, A SAR is induced by trees in close proximity. There is little point in planting companion trees at a distance where they are unlikely to induce a SAR.
It could be argued that the best companion ( in this context a "companion" is a competitor) is another tree of the same species. There are several advantages in planting blackwoods in groups, at final spacing (14):
- induction of a SAR.
- an improved selection ratio.
- it ensures even spacing of final crop trees.
- a reduction in cost
To avoid crown-shy growth , the trees in each group should be thinned to a final crop tree when they reach 6 to 8 metres in height.
I have used group planting for blackwood for over 30 years ( 4 per group, 1 to 2 metres apart within the group, and 7 to 8 metres between groups). I have a strong impression that this induces a SAR, but have not formally tested it against controls. I think there might be a case for a formal trial, comparing group planting with open-grown trees that are even spaced. This could involve a study of the architecture and juvenile foliage retention, and include the use of a spectrophotometer. The Pirongia study suggests an optimal spacing within the groups of about 1 metre.
Genetic gain
The search for genetic gain by selection from plus trees has been very successful in forestry. However in blackwood a problem with plus tree selection is that some of the attributes that are sought are strongly influenced by environmental rather than genetic factors. In a study at Pirongia (17), of genetically identical blackwoods grown from root cuttings from the same parent tree, we found great variation in vigour and form, although wood colour seems to be consistent. This suggests a powerful influence of microsite variation.
As a method of levelling the environmental playing field, I can suggest a method that might be useful, and worth a trial. As referred to, blackwoods from high rainfall coastal provenances retain juvenile characteristics longer than those from dry inland sites, and develop better growth rates and form. It is likely that similar variation is present between trees within the same provenance. This might form the basis for a trial of seedling selection in the nursery, where they are grown under uniform conditions free from environmental influence, and where variation between seedlings is likely to be an expression of genetic variation. It might be expected that that seedlings that retain juvenile foliage would perform better in the field.
This could be tested in a trial of trees from the same provenance in which seedlings showing strong retention of juvenile foliage are selected , and compared in the field with a group that show early conversion to phyllodes. If the first group showed enhanced performance, this might be useful when screening trees for a breeding population.
Hormone study
Nicola's study showed a growth response to giberellin, which produced height increment and a reversion to juvenile foliage, although with loss of apical dominance. It has been subsequently shown that auxin is involved in the SAR, and is likely to be the missing link in controlling apical dominance.
It might be of interest to repeat the study ( perhaps a student topic), using different combinations of auxin and giberellin. Whether this might have practical application is doubtful, but if a suitable combination could be shown to induce juvenile foliage combined with strong apical dominance, it might be worth a trial designed to test whether a spray application at the time of planting in the field might influence early growth form.
Acacia psyllids and phase change
Acacia psyllids are endemic to Australia, and are prevalent in blackwood plantations in New Zealand. They feed on and damage growing shoots, and the honeydew they produce attracts sooty mould fungi. It has often been assumed that they are responsible for malformation in blackwood. My own view is that psyllid populations are closely linked to phase change and growth periodicity. What follows are some anecdotal observations :
- psyllids do not attack healthy juvenile foliage. They are usually absent from blackwoods in their juvenile growth phase in the first summer, and appear when foliage converts to phyllodes from the second year.
- When different age classes are mixed on the same site where psyllids are active, the trees in juvenile foliage are not affected. Similarly, when stem shoots bearing juvenile foliage appear on older trees, the juvenile foliage shows continuous growth and is not damaged. At the same time, older shoots bearing phyllodes are attacked as they terminate their growth periods.
- as blackwoods shift from continuous into periodic growth they terminate their growth periods by aborting their shoot tips. This commonly occurs in December, and after resuming growth, again in autumn. When growth resumes from sub-terminal shoots, this causes loss of apical dominance, and the result is branching and malformation. Shoot tip abortion is not an accident, or a consequence of damage by psyllids, but a natural process, and it provides the tree with a mechanism for developing a spreading crown when exposed to light.
- we have carried out trials in which psyllids were controlled by chemical sprays. Both the treated and control trees aborted their shoot tips. This process was delayed when psyllids were controlled, allowing a longer period of extension growth. This allowed an increase in extension growth of up to 40% when psyllids were controlled , compared with unsprayed controls. However both groups showed a similar pattern of malformation.
- Psyllids appear to time their periods of maximal activity to changes within the shoots as they approach the end of their growth periods. Perhaps they are attracted by an increase in nutrients, or chemical signals that occur at that time.
- In the course of our giberellin study, we increased the dose of hormone to a level of toxicity. At that point the juvenile shoots showed signs of stress, and they were then attacked by psyllids. This suggests that juvenile foliage is not inherently resistant to psyllids, but is protected by its habit of continuous growth.
- acacia psyllids are not present in Chile. This might partially explain the strong growth apparent in young blackwoods in Chile.
- in a trial at Pirongia of blackwoods from over 60 provenances representing the natural range in Australia, none showed apparent resistance to psyllids.
- if an effective biological control agent for psyllids could be found, it could be expected to improve the growth performance of blackwoods The recently released Southern ladybird (Cleobora mellyi) might have an effect. They have been successfully released on my Pirongia plantation.
- an attempt to control psyllids by chemical sprays would be costly, time-consuming, and environmentally unsound. I have attempted limited control by applying a brief spray application to the terminal shoot tip from a back pack while form pruning in late spring, but have not tested the effect against controls. It might be worth a trial to determine whether this produces a useful gain in extension growth.
Totara and SAR
Totara, like blackwood, has a highly developed shade avoidance response consistent with a light-demanding species. When open grown, it has a disorderly growth habit, with poor apical dominance and multiple branches. When closely planted, or regenerating in close competition, it produces a straight stem and an orderly branch sequence.
It is easy to correct malformation in blackwood by regular form pruning. However I have found totara to be much more difficult, and my attempts to do so have been unrewarding.
A SAR can be produced by close planting, but this is clearly a costly option. As an alternative, there might be a case for group planting as an alternative to planting in rows. From a casual observation of totara regenerating at random on a Northland farm, I have an impression that when grouped by chance in close proximity they appear to show a SAR, with an improvement in stem form . It might be of interest to conduct a small trial. I would suggest a spacing of about one metre between trees within groups of 2 or 4 trees.
As an alternative, the use of tree shelters with totara would be likely to cause severe instability.
Appendix.
Tree Shelter Trial.
In September 2013 I set up a small trial to test the effect of low R shelters on blackwood seedlings.
I planted 30 seedlings on open ground at 4 metre spacing, with a generous release spray to eliminate reflected light. 24 seedlings were marked in pairs, in which the trees were closely matched for vigour, and the ratio of juvenile foliage to phyllodes. To further level the playing field, all seedlings were coppiced to 5 cm. 80 cm shelters were constructed, 12 of low R (Lee 121) filters, and 12 of clear plastic. These were placed alternately over corresponding seedlings in each pair. The remaining 6 seedlings were planted in the open.
The trees were examined at 2 week intervals to measure height, the proportion of juvenile to adult foliage, and branch size. They were also checked for shoot tip abortion (STA), which is closely linked with malformation.
Early Results.
Growth Rate. The trees in low R filters showed accelerated height growth compared with those in clear plastic. Of the first 9 to emerge from the top of the shelters, 8 were in the low R group. After emerging, there was some convergence in growth rates, but by April there was still a clear distinction ( mean height difference 26%).
Foliage Type. The foliage within the low R shelters was predominately juvenile (11/12). 2 of these converted to phyllodes after emerging from the shelter. Within the clear shelters, phyllodes were predominant (9/12).
Branching. Seedlings dominated by phyllodes had larger branches. Several of these showed STA, with loss of apical dominance, and early malformation.
Open grown seedlings. 5 of these produced early phyllodes, and showed slow growth, STA, and malformation. The other, in striking contrast, and for no apparent reason related to site, produced sustained juvenile foliage to a height of 2 metres, before starting to convert to phyllodes.
Summary. In this small trial, low R shelters delayed the conversion of juvenile foliage to phyllodes. This was associated with faster height growth, smaller branches, and absence of STA. The numbers are small, and would need to be validated by a larger trial, preferably on more than one site. There would also need to be a longer period of study to determine whether the effect is sustained, and whether it is practically and economically viable.
Differing roles in juvenile foliage.
From the above it appears that blackwoods respond to low R light reflected from adjacent vegetation by delaying the conversion from the juvenile to adult growth phase. This gives them a competitive advantage in low light conditions, and would seem to be one of the mechanisms of the nurse effect. This is likely to be true in other species that occupy a similar ecological niche.
What of species that are shade-intolerant? On a day back in 1997 I was on Pirongia with John Bathgate, who was collecting data on our trial blackwoods. He had been measuring photosynthetic rates with a portable device that measures stomatal conductivity. As expected, the phyllodes showed a stronger photosynthetic response in sunlight than the juvenile leaves.
On the way back we passed a group of 3 year old E. nitens, which had the typical mixture of juvenile and adult leaves. Out of curiosity, John measured the stomatal conductivity on the adult leaves. As expected, these showed high photosynthetic activity. He then tested the juvenile leaves. If these had been blackwoods, the result would have been a lower value. In fact, the opposite was true : the level was near the top of the scale. John's comment was that the juvenile leaves were "turbocharged".
If the juvenile leaves in blackwood are an adaptation to low light in a crowded environment, their equivalents in E. nitens are photosynthetic turbochargers, serving the needs of an alpha species that takes no prisoners and dominates its competitors in the pursuit of light.
Among the heterophyllic New Zealand species, the juvenile growth phase is commonly associated with a divaricating growth habit. Of the competing explanations for this, the Moa-defence theory is the most interesting, although for obvious reasons unprovable. Other contenders include an adaptation to harsh environments. Clearly the juvenile growth phase can fulfil different roles among species, including responses to different light regimes, environmental exposure, and perhaps predation from extinct megafauna.
REFERENCES
1. Casal, J.J ; Smith, H. 1989 : The function, action and adaptive significance of phytochrome in light-grown plants. Plant ,Cell, and Environment 12: 855-862.
2. Warrington, L.J. ; Rook, D.A. ; Morgan, D.C. ; Turnbull, H.L. 1988 : The influence of simulated shadelight and daylight on growth, development and photosynthesis of Pinus radiata, Agathis australis, and Dacrydium cupressicum. Plant, Cell, and Environment 12 : 343-356.
3.Ballare, C.L. ; Sanchez, R.A. ; Scopel, A.L. ; Casal, J.J. ; Ghorsa, C.M. 1987 : Early detection of neighbour plants by phytochrome perception of spectral changes in reflected sunlight. Plant, Cell, and Environment 10 : 551-557.
4. Gilbert,R. ; Seavers, G.P. ; Jarvis,P.G. ; Smith, H. 1995 : Photomorphogenesis and Canopy dynamics. Phytochrome-mediated proximity perception accounts for the growth dynamics of Populus trichocarpa x deltoides "Beaupre". Plant, Cell, and Environment 18 : 475-497.
5. Forster, M.A. ; Bonser, S.P. 2009 : Heteroblastic Development and shade avoidance in response to blue and red light signals in Acacia implexa. Photochemistry and Photobiology 85 ; 1375-1383.
6. Brodribb,T ; Hill,R.S. A physiological comparison of leaves and phyllodes in Acacia melanoxylon. Australian Journal of Botany 41; 293-305.
7.Salter, M.G. ; Franklin,K.A. ; Whitlam,G. 2003 : Gating of the rapid shade-avoidance response by the circadian clock in plants. Nature 426 ; 680-683.
8.Forster,M.A. ; Ladd, B. ; Bonser, S.P. 2010 : Optimal allocation of resources to shading and neighbours in the heteroblastic species, Acacia implexa. Annals of Botany Dec.6.
9.Farrell, T.P. ; Ashton,D.H. 1978 : Population studies of Acacia melanoxylon. Variation in seed and vegetation characteristics. Australian Journal of Botany 26 ; 241-257.
10. Bathgate,J. ; Brown, M. 1996 :Effect of shelter on early growth and form of Tasmanian blackwood in the Waikato region. AMIGO newsletter, No.3.
11.Borchert, R. 1965 : Giberellic acid and rejuvenation of apical meristems in Acacia melanoxylon. Naturwissenschaften 52 ; 65-66.
12.Stamm, P. ; Kumar,P. 2010 : The Phytochrome signal network regulating elongation growth during shade avoidance. Journal of Experimental Botany 11 : 2889-2903.
13. Pierik, R. ; Djakovic-Petrovic,T. ; de Wit, M. ; Voesenek, L. 2009 : Auxin and Ethylene regulate elongation responses to neighbour proximity signals independent of giberellin and DELLA proteins in Arabidopsis. Plant Physiology 149-4 : 1701-1712.
14.Nicholas, I.D. ; Brown, I.D. 2002. : Blackwood. A Handbook for growers and end users. Forest Research Bulletin No 225.
15. Brown, I.D. 1998 : Tree Shelters. How good are they? New Zealand Tree Grower 18 ; p3.
16. Ledgard, N ; Giller, M. 1999 : Form Pruning deciduous hardwoods. New Zealand Tree Grower 20 ; 36
17. Brown,I.D. ; Nicholas,I.D. :NZFFA Conference Proceedings, 2007
Disclaimer: Personal views expressed in this blog are those of the writers and do not necessarily represent those of the NZ Farm Forestry Association.
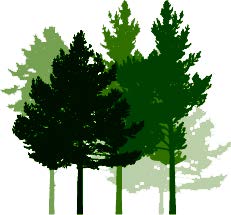
No posts yet
Add a post